Reversible Solid Oxide Fuel Cell (R-SOFC)
To enable the generation of efficient, low-cost electricity and hydrogen.
The solid oxide fuel cell (SOFC), as a fuel cell mode, is an electrochemical device that converts chemical energy of a fuel and oxidant directly into electrical energy. It can also function in water electrolyzer mode to produce hydrogen with a byproduct of oxygen. This configuration is known as a solid oxide electrolysis cell (SOEC) or reversible solid oxide fuel cells (R-SOFC)
The R-SOFC Program is also developing the synergistic solid oxide electrolysis cell (SOEC) technology. Electrolysis is a process that splits hydrogen from water using an electric current. SOEC systems offer a potentially attractive option for producing hydrogen because of high efficiency and system flexibility. In addition to the development of standalone SOEC systems, developers are exploring the potential to use both the SOEC and SOFC in a single hybrid device (R-SOFC) in order to produce electricity during times of high demand (high value) and to produce hydrogen during times of off peak demand (low cost). The hydrogen produced during off-peak demand could, for example, later be used in electricity generation and this makes the SOEC system a key component in enabling the wider adaption of distributed renewable power sources such as wind and solar.
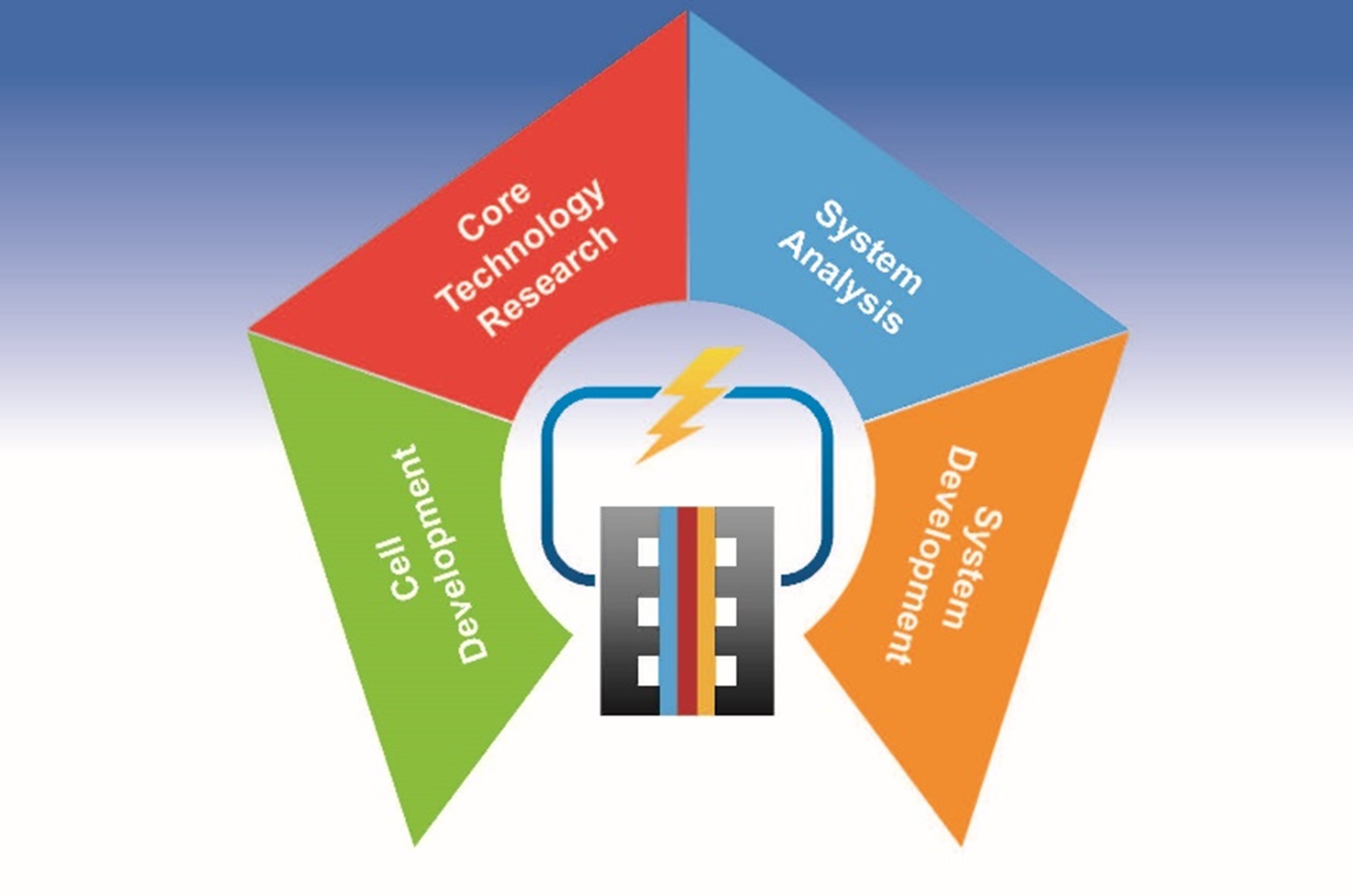
The NETL Reversible Solid Oxide Fuel Cell Program maintains a portfolio of research and development (R&D) projects that address the technical issues facing the commercialization of R-SOFC technologies and pilot-scale testing projects intended to validate the solutions to those issues. To successfully complete the maturation of the R-SOFC technology from its present state to the point of commercial readiness, the Program’s efforts are channeled through three key technologies, each of which has its respective research focus.
SOFC Operating Principle – click here to see how a solid oxide fuel cell works.
SOEC Operating Principle – click here to see how a solid oxide electrolysis cell works.
Key Technology Areas
The R-SOFC Program R&D efforts apply to three key technology areas for SOFCs and SOECs: Cell Development, Core Technology, and Systems Development.
Cell Development
Research is focused on the cell-related technologies critical to the commercialization of R-SOFC technology. The components of the R- SOFC - the anode, cathode and electrolyte – are the primary research emphasis of this key technology. The electrochemical performance, durability, and reliability of the reversible solid oxide fuel cell are key determinants in establishing the technical and economic viability of R-SOFC Power Systems. Thus, the R-SOFC Program maintains a diversified portfolio of cell development projects that are focused on improving electrochemical performance and cell power density, reducing long term degradation, developing more robust cells, and cost reduction. Additional research projects include evaluation of contaminants, advanced materials, materials characterization, advanced manufacturing, and failure analysis. Program participants include academia, national laboratories, small businesses, the NETL Research & Innovation Center, and other R&D organizations. The portfolio maintains a mix of near-, mid-, and long-term R&D projects at bench- and laboratory-scale.
Core Technology
This key technology conducts applied research and development on technologies – exclusive of the cell components – that improve the cost, performance, robustness, reliability, and endurance of R-SOFC stack or balance-of-plant (BOP) technology. Projects in the Core Technology portfolio focus on interconnects and seals, identify and mitigate stack-related degradation, develop computational tools and models, and conduct laboratory- and bench-scale testing to improve the reliability, robustness, endurance, and cost of stacks and BOP components, respectively. Program participants include academia, national laboratories, small businesses, the NETL Research & Innovation Center, and other R&D organizations.
Systems Development
This key technology maintains a portfolio of projects that focus on the research, development, and demonstration (RD&D) of R-SOFC power systems. Project participants (industry teams) are independently developing unique and proprietary R-SOFC technology suitable for either syngas- or natural gas fueled applications. The industry teams are responsible for the design and manufacture of the fuel cells, integration of cells hardware development, manufacturing process development, commercialization of the technology, and market penetration. These developers also focus on the scale up of cells and stacks for aggregation into fuel cell modules and the validation of technology. This key technology also supports laboratory-scale stack tests, proof-of-concept systems, and pilot-scale tests. The multi-industry team approach not only provides technology diversification but also offers insurance against business environment risk, reducing program dependency on a single developer. The industry teams have the opportunity to determine relevant R&D topics based on their design-specific experience and needs and are held to a common set of performance and cost metrics.
Also within the Systems Development key technology is a portfolio of projects focused on innovative concepts. These projects conduct bench-scale R&D on innovative R-SOFC stack technologies that have the potential to significantly decrease the cost of R-SOFC power systems by leveraging advancements in lower-cost materials, advanced manufacturing methods, and/or alternative architectures.
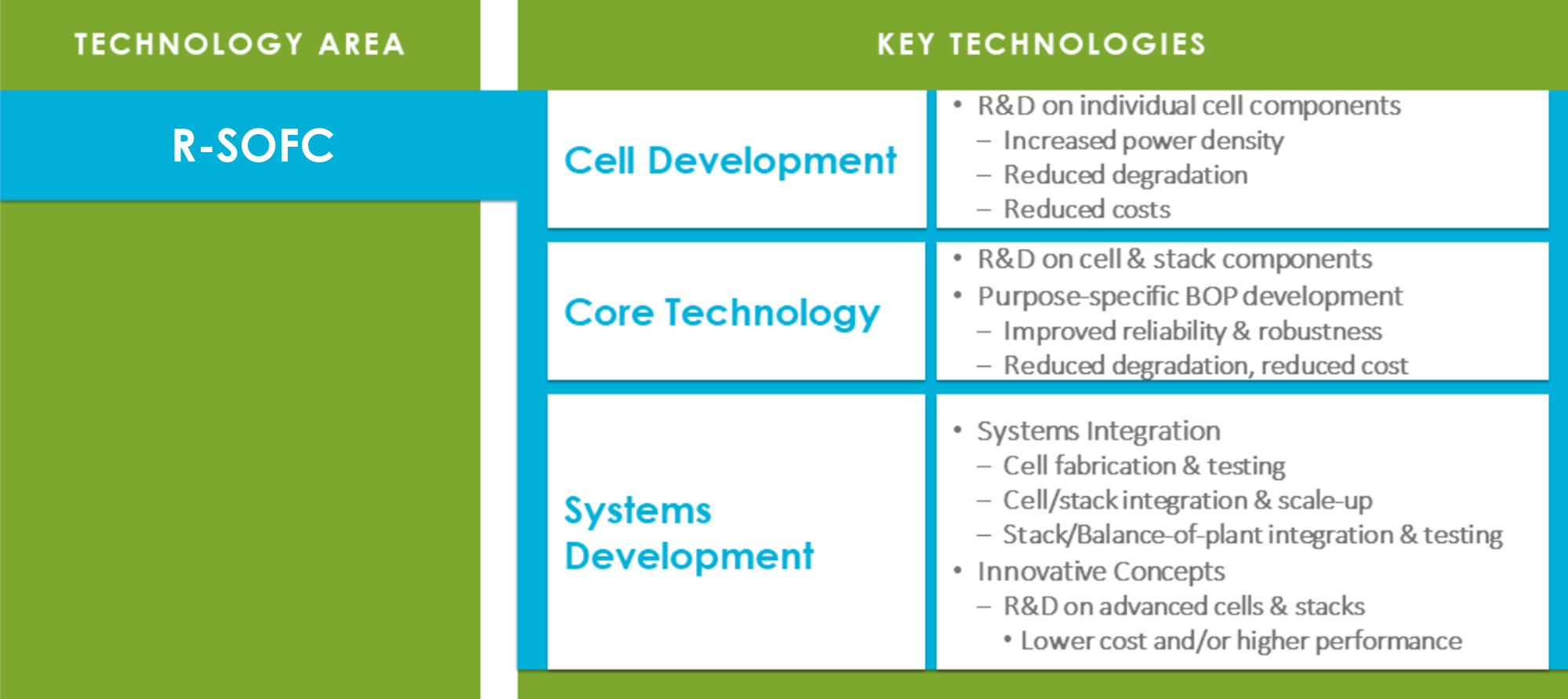
Explore the Site
NETL implements this effort as part of DOE’s Advanced Energy Systems Program.